Orbea variegata (formerly Stapelia variegata), about 10cm across. Part of the milkweed family, would you believe.
Thursday, December 30, 2010
Friday Flora
Orbea variegata (formerly Stapelia variegata), about 10cm across. Part of the milkweed family, would you believe.
Wednesday, December 29, 2010
Encroaching decrepitude

It's also been noted that I look more professorial.
Monday, December 27, 2010
Monday Musical Offering
Friday, December 24, 2010
The Microwunderkammer pledge
No posting on this blog, Microwunderkammer, will ever be typed or texted while using a urinal.
Monday, December 20, 2010
Monday Musical Offering

Saturday, December 18, 2010
Well, we liked it
I can talk a little about the performance--Tetzlaff clearly can do whatever he wants on the violin, and some of what he did that night was really magnificent. He communicated the music clearly, and with enough freedom that it became personal. I particularly enjoyed his slower movements. I did take issue with some of the faster movements, especially the Gigues with which the suites end. These are supposed to be fast, but they are dances and should maintain their distinctive triple beat. However, Tetzlaff played them really really fast--so fast that they pretty nearly lost their beat, and just became a rapid-fire string of notes. However, on the whole, I really enjoyed the concert.
There were people there who did have standing to be critics; seated behind us was an older woman who also had the score with her. She worked as a violin teacher, and she said of his faster movements that "that's not the way I learned it. Perhaps he had a plane to catch."
Friday, December 17, 2010
Thursday, December 16, 2010
Buddy Breathing
We all need to breathe. This is true for us humans, and it’s true for most microbes. However, many microbes have different approaches to breathing. A new discovery from Derek Lovley at University of Massachusetts reveals surprising flexibility in microbial respiration—and how a couple of bacteria can cooperate and evolve to meet a challenge to their survival.
When we breathe, we’re consuming oxygen; we need this oxygen to oxidize our food, which is mostly carbon and hydrogen (think “carbohydrates”). We often think of oxidation as simply “reaction with oxygen”. After all, we are taking the carbon and hydrogen we eat and making it into carbon dioxide and di-hydrogen oxide (or water, for the less nerdy). However, there is a more precise definition of oxidation that appeals to chemists and microbiologists. Oxidation is the loss of electrons by an atom; since the electrons have to go somewhere, they are accepted by another atom, which we say is getting reduced.
When we respire (which is why we breathe), we are taking electrons away from the carbon and hydrogen in our food, and giving those electrons to oxygen. We get energy from this process, which we use to make the ATP that powers the cell. But Bacteria and Archaea have figured out how to take electrons from carbon and hydrogen and give them to atoms other than oxygen: sulfur, iron, arsenic, and all manner of other atoms. They don’t necessarily get as much energy out of these processes, but it’s better than dying if no oxygen is available.
One example of this type of anaerobic (no-oxygen) respiration is seen in the Bacterium Geobacter metalloreducens, which, as its name suggests, reduces metals. When it respires, it “eats” ethanol, taking away its electrons, and “breathes” metals such as iron, giving them electrons and producing rust. Another example of this type of respiration is found in the related bacterium Geobacter sulfurreducens. It can’t eat ethanol, but it can eat hydrogen. It can’t “breathe” oxygen or iron, but it can give electrons to sulfur (as the name suggests) or the compound fumarate.
Whether or not oxygen is used for respiration, the basic outline of the process is the same: electrons are plucked off of the food, and go through a series of intermediate carrier molecules before they end up at the terminal electron acceptor. The whole process takes place in the cell membrane: the electrons on their carriers never leave the cell until they are handed to the terminal electron acceptor. As the electron is passed from carrier to carrier, it releases energy, which is used to make ATP.
The outline of this process is the same for us, and for Geobacter metalloreducens and for its cousin sulfurreducens. However, metalloreducens and sulfurreducens are specialized for particular combinations of food and electron acceptor: metalloreducens likes ethanol and iron, while sulfurreducens likes hydrogen and fumarate.
But what happens if you put these two organisms in an environment with food for only one, and an electron acceptor for only the other—ethanol and fumarate? It would seem that metalloreducens could take electrons from ethanol, but not get rid of them, while sulfurreducens could give electrons to fumarate—if only it could obtain some electrons from somewhere!
Fortunately, these two different types of cells can cooperate. Geobacter metalloreducens can get rid of electrons from ethanol—it just won’t get much energy out of the deal. Water, it turns out, is mainly H2O, but always has some OH- and some H+; metalloreducens can give those electrons from oxidizing ethanol to H+, making hydrogen gas. This works out nicely, since sulfurreducens can “eat” the hydrogen, and give the electrons to fumarate. So, even though each member of the partnership only gets half as much energy, it’s better than getting no energy at all.
This type of collaboration is called “syntrophy,” and it’s actually fairly common in the microbial world. One organism respires, producing hydrogen; the next organism respires, “eating” that hydrogen. Each organism has a complete respiratory system, but the two respiratory systems are dependent upon each other. Syntrophy occurs in your own personal digestive system, in which one organism makes hydrogen, giving electrons to another organism, which uses them to make the methane that makes our farts flammable. Up till now, when syntrophy has been studied, it has always involved hydrogen as the electron carrier. However, Lovley’s group introduced an interesting complication to this process.
Lovley’s group mutated sulfurreducens so that it absolutely can’t eat hydrogen. They then started the experiment again, giving a mixture of metalloreducens and sulfurreducens nothing but ethanol to eat and nothing but fumarate to breathe. Given that the options for these populations of cells were (a) evolve or (b) die, it’s unsurprising that option (a) was chosen.
What was surprising was the way that evolution worked. Rather than jury-rigging a way for sulfurreducens to eat hydrogen, evolution chose a method that involved the direct transfer of electrons from metalloreducens to sulfurreducens. I mentioned that when a cell respires, the electron gets shuttled from one carrier molecule to another as it moves from the “food” to the electron acceptor. These carrier molecules stay in the cell membrane, and respiration happens strictly within the confines of the cell.
What Lovley found was a mutant form of sulfurreducens that produced electron carriers that could be exported to cells of metalloreducens, pick up electrons, and then shuttle back to sulfurreducens. The sulfurreducens cell had to make filaments for these carriers to travel along, and had to grow next to metalloreducens—but one part of respiration was happening in one cell, and the other part of respiration was happening in another cell. This is syntrophy, but without using hydrogen to transfer the electrons. Electrons were flowing directly from one cell to the other.
Being thorough, Lovley’s group made sure that they were seeing electrons moving directly between the two cells. They checked to see if hydrogen was being exchanged—it wasn’t. They found the genes in sulfurreducens that had mutated to allow this to happen, and deleted them—and growth stopped. They looked for electrical current between cells—and found up to four microamperes of electron flow. They looked at the cells using various forms of microscopy, and found that they grow in close contact…
(this is a clump of cells, with metalloreducens stained green and sulfurreducens stained red. They can't grow separately, like the cells in a normal syntrophic relationship.)
…and they used the much higher magnification of electron microscopy to show that sulfurreducens made a web of filaments that connected it to metalloreducens cells, and that electron carriers (visible as dark spots) could move along those filaments:
This has never been seen before—respiration has always been contained within one cell. But now, we know that the process of respiration can be completely shared between two cells, a sort of bacterial “buddy breathing.” One cell oxidizes food (metalloreducens taking electrons from ethanol) and the other reduces an electron acceptor (sulfurreducens giving electrons to fumarate). This kind of metabolic sharing allows both cells to survive in an environment that could not sustain each individual, and so gives life the ability to exploit ever more of the earth’s environment. It’s also a nice example of life’s seemingly endless creativity—what Daniel Koshland calls “improvisation”—using mutation and selection to try out novel solutions to novel problems.
Zarath M. Summers, Heather E. Fogarty, Ching Leang, Ashley E. Franks, Nikhil S. Malvankar, and Derek R. Lovley (2010). Direct Exchange of Electrons Within Aggregates of an Evolved Syntrophic Coculture of Anaerobic Bacteria. Science 330: 1413-1415.
Koshland, Daniel (2002). The Seven Pillars of Life. Science 295:2215-6.
Tuesday, December 14, 2010
Twitchy
The most common image that people have of how bacteria move is of a little bug swimming along using its flagella. So busy!
But the bacteria have other means of getting around—one of the more amusing ways of moving is called “twitching motility.” Cells that move by twitching motility don’t have a flagellum. Instead, they produce a thing called a "pilus." This is kind of like the telescoping radio antenna you see on a car: it’s a thin shaft of protein that can extend from the cell, then retract back--here's Pseudomonas pulling in some pili:
The end of the pilus has a sticky protein on it, so to continue our metaphor, the pilus is like a telescoping antenna with a blob of crazy glue on the end. A twitching cell extends its pilus, sticks it to something, then draws it back in. Here's Pseudomonas grabbing onto a hair, then pulling itself along:
Rather than smoothly swimming, the motion is herky-jerky. Or, twitching. So, it's called twitching motility!
This is how I’ve always pictured twitching motility: a cell lying down, and moving in a linear motion. But, what if the cell is standing on end? If it just made one pilus, it would pogo up and down. But if it made a few pili, it would sort of stagger—go up, then go down a little off to one side; go up, then go down a little off in another direction, depending on how poorly synchronized the pili were. A twitching cell standing on end would “walk,” but it would be a drunkard’s walk.
It turns out that there are situations where a drunkard’s walk is actually very useful. An army of drunkards, all starting at the same place, will effectively “explore” every nook and cranny of an area. If the “drunkards” are twitching bacteria, standing on end, then a population of bacteria can effectively, if blindly, explore every bit of an area, and if there’s a particularly good place to set up shop, at least one individual bacterium is bound to find it (panel A).
In contrast, the bacteria who are “lying down” and moving by twitching motility will travel farther, but won’t cover an area as thoroughly (panel B).
This isn’t just an amusing vision of staggering bacteria—a set of experiments led by Gerard C. L. Wong showed that certain types of bacteria actually use this method to effectively explore an area. Using microscopes rigged with cameras and computers, he was able to track individual bacteria; he found that the “staggerers” were much better at covering an area than the twitchers who were lying down, or those lacking pili altogether.
The bacteria that lacked the ability to make pili never really explored their environment, so they ended up crowded—a situation that makes it hard for them to get the food they need.
Bacteria that could make pili and walk around after dividing were nicely spaced out, with plenty of elbow room—and access to food.
The need to get up and explore seems to be built in to the bacterial program: Wong’s group watched hundreds of individual bacteria divide, and most often division resulted in one cell that was lying down and another cell that was “standing,” ready to get up on its pili and stagger around. If things were just due to chance, you'd expect far more freshly divided bacteria to be lying down--not the most efficient way to disperse. It seems that roving is in the genes.
This is not an earth-shattering discovery. However it does provide some insight into how bacteria explore and exploit their environment, and how wanderlust seems to be genetically programmed. It also provides the amusing mental image of a cell, twitching and staggering around like a Dickensian drunkard looking for a place to sleep it off.
Maxsim L. Gibiansky, Jacinta C. Conrad, Fan Jin, Vernita D. Gordon, Dominick A. Motto, Margie A. Mathewson, Wiktor G. Stopka, Daria C. Zelasko, Joshua D. Shrout, Gerard C. L. Wong (2010) Bacteria Use Type IV Pili to Walk Upright and Detach from Surfaces. Science 330:197.
The movies are from Howard Berg's lab's excellent website.
Alea jacta est!
Now, time to write the letters of recommendation.
Wednesday, December 8, 2010
"Will they re-write the textbooks?"
The authors really needed to do a bit more chemistry--as they noted, the cells still contain phosphorus, and some patient calculations (by people who weren't busy with the final week of the quarter) suggested that the amount of phosphorus is enough to sustain a somewhat unhappy cell. Indeed, that's the most vexing thing--even after many generations of passage, there was still phosphorus in all the cells, leaving a lingering doubt throughout the whole project. More rigorous chemistry procedures are required. It's necessary to do the hard work to make really phosphorus free media, and really really purify the DNA and small-molecule fractions so there's no chance of random cellular arsenic contaminating an otherwise normal sample. So, there's stuff to be cleaned up, and it can and should be cleaned up. I think that the authors' claim is still possible, but more proof is necessary.
It's been interesting to see how various blogs have treated the story--generally, not nicely (see here, here, and here for a few, including John Roth and Jonathan Eisen here at Davis). Part of the issue has been the lack of necessary rigor. But I think people have been especially eager to hit this story because of how it was presented: this was the classic case of science by press release, with a one-week-long drum roll leading to the reveal. That's just asking for it. While newspapers love science by press release (it saves thinking), most bloggers (who are more scientist than journo) hate hate HATE science by press release.
UPDATE--14 December. I just spent the weekend at the West Coast Bacterial Physiologists Annual Meeting. This was not mentioned once--an index of how much regard it has gotten.
Monday, December 6, 2010
Monday Musical Offering better late than never edition
Friday, December 3, 2010
OMG! ARSENIC EATING MICROBES!!1!
It’s quite a roll-out: first, NASA’s astrobiology program announces a very important press conference coming in one week. There is all kinds of speculation, some of it quite fervid. Finally, the news. No Martians, no radio signals from Tau Centauri. Instead, a more mundane headline in the NY Times says “Microbe Finds Arsenic Tasty; Redefines Life.”
The actual science is interesting, though it doesn’t quite live up to the hype (surprise!). While the the hype is about “redefining life” and “stretching the possibilities for extra-terrestrial life,” the real interest lies in a demonstration of the power of selection and what down-to-earth microbes can do.
Here on earth, microbes (and humans, and every other living thing) have to grow—they take in atoms from their environment and convert that “dead” matter into a part of a cell. Every living thing uses pretty much the same ingredients: Carbon, Hydrogen, Nitrogen, Oxygen, and Phosphorus, with a sprinkle of other elements Why only those particular elements? Carbon is neat, but could it be substituted with an element from the same column on the periodic table, such as Silicon? No. Even though it shares some characteristics with carbon, it just doesn’t have the same wonderful reactive repertoire.
This has not stopped speculation about the other ingredients found in a cell; Felisa Wolfe-Simon at the NASA Astrobiology Institute and her coworkers wondered if the phosphorus found in the cell could be replaced with a similar atom on the periodic table. However, the element most similar to phosphorus on the periodic table is Arsenic.
As the headline suggests, Wolfe-Simon found—or more correctly, evolved—a microbe that can almost completely replace its phosphorus with arsenic. In this cell, arsenic behaves similarly enough to phosphorus that it grows reasonably well. However, despite the headlines, very little adjustment need be made to the definition of life. Some definitions of life hold that “life is mainly made of the elements Carbon, Hydrogen, Nitrogen, Oxygen, and Phosphorus.” So, that can be revised to read “…and Phosphorus (or Arsenic).” Ho hum.
The most interesting thing about this report was its application of selection. Natural selection is one of the driving forces behind evolution—from a varied initial population, the environment selects those individuals who are best able to survive and reproduce, and allows them to do so. The rest don’t make it. It’s very simple, but amazingly powerful. You can do this in the laboratory: start with a varied initial population, select only those individuals who have a certain trait (say, the ability to survive exposure to Arsenic), and allow only them to reproduce; the rest die.
As my mentors taught me, “you get what you select for.” If you start with a very large and very varied population, and slowly ratchet up the selection pressure, you can select for, and evolve, almost anything. In fact, something very similar to this recent study was accomplished over a decade ago, using slightly cleverer techniques.
A biology textbook might refine the above definition of life by mentioning proteins, and how they are all made of the same 20 amino acids, just in different arrangements. There is a genetic code, in which each of the 20 amino acids is specified by three nucleotides strung together in DNA. There are four different nucleotides (called A, G, C, and T), so there are 64 possible three-nucleotide combinations. 61 of these specify the 20 amino acids (there is obviously some redundancy), and three specify the end of a protein. You probably saw this table back in high school:
Starting in the 1980s, researchers (most notably Peter Schultz) tried to redefine life: they wanted to make proteins that had different, new, non-biological amino acids in them. To do this, they had to somehow persuade the cells to change the way they decoded their genetic information. Essentially, they had to convince a cell that “UAG” no longer should be decoded as “stop”, but rather as the bizarre new amino acid Propargyloxyphenylalanine. The cell, you might imagine, required some strong persuasion—and this is where selection came in.
Schultz’s group cleverly placed a gene in the cell for chloramphenicol resistance—if this gene were properly expressed, the cell could survive exposure to the antibiotic chloramphenicol. However, this gene was modified: it could only be properly expressed if “UAG” were translated as Propargyloxyphenylalanine. If UAG were used normally, as “stop”, the cell would stop making the protein, and die. That’s selection for you—and it allowed Schultz to find the very rare cell that had mutated so that it could pull of this trick. All he had to do was look for cells that survived chloramphenicol exposure.
Schultz used selection again to make sure hat he had succeeded; there was, after all, the possibility that the cell sometimes translated UAG as Propargyloxyphenylalanine, and sometimes translated UAG as some other amino acid. So, he cleverly placed another gene in the cell that encoded a weird protein called “barnase.” If the cell makes even a little bit of barnase, the barnase will kill the cell. The gene that Schultz used also had a UAG in it, but in this case, functional barnase would only be produced if UAG were translated as anything but Propargyloxyphenylalanine. Again, selection allowed Schultz to find the very rare cell that only translated UAG as Propargyloxyphenylalanine—selection killed every other cell. Again, all he had to do was look for the surviving cells.
So, you get what you select for—no matter how rare or absurd. Using the power of selection, Schultz and others have evolved cells that use more than 70 different, completely non-biological amino acids in their proteins—a pretty thorough redefinition of this aspect of life. How does this relate to the Arsenic story? Selection. Wolfe-Simon selected for the ability to use Arsenic in place of phosphorus, and you get what you select for.
She started with microbes that were already pretty tolerant of arsenic. They are not from a very unusual family—they are proteobacteria, a family name as bland among bacteria as “Smith” among Americans. However, these had been recovered from Mono Lake in California, an environment with unusually high concentrations of arsenic. In the lab, the cells were grown with increasing amounts of arsenic and decreasing amounts of phosphorus. These conditions selected for the ability to replace phosphorus with arsenic, so cells that could do this would grow and reproduce. Those cells that could not (and these were the vast majority) simply died. The selection was made harsher and harsher, so that eventually cells were growing in as close to phosphorus-free conditions as could be managed. Selection found the rare cells that learned to use arsenic, and all Wolf-Simon had to do was look for the survivors. Over the course of just a few generations, Wolfe-Simon forced the evolution of an Arsenic-using bacterium (figure C).
This is a rather extraordinary claim, and as Carl Sagan noted, extraordinary claims require extraordinary evidence. So, the bulk of Wolf-Simon’s report consists of proofs that these cells are chock-full of arsenic and very low in phosphorus. It’s bizarre—virtually everything that is usually has phosphorus has arsenic. So DNA and RNA have a sugar-arsenate backbone; ATP is now Adenosine tri-Arsenate; NADH has Arsenate rather than phosphate; the membrane phospholipids are now arseno-lipids, and so on. There’s a hint of phosphorus left. (One thing that needs to be cleared up is just how much phosphorus there is left, and where it is.) These cells (figure C) are strikingly different in aspect from their immediate “wild” ancestors (figure D). The Arsenic-incorporating cells are larger and somewhat irregularly shaped. A cross-section electron micrograph shows that much of their volume is made up of storage vacuoles absent in their ancestors (figure E; note that the magnification is five times greater). These may be for storing excess arsenic, or protecting some cellular machinery from arsenic.
These are not exceptionally happy cells, though: it takes them almost two days to grow and divide. One can almost hear them sighing with relief when the arsenic is taken away and replaced with phosphorus; their growth rate almost doubles.
Like any good study, this one raises lots of new (and previously undreamt-of) questions. The ancestors of these cells were isolated from an arsenic-rich environment—so, how much arsenic (if any) is there incorporated into these cells in the wild? How much actual genetic difference is there between the laboratory-grown cells and wild cells? Could one evolve arsenic-using cells from other cells in the same environment, or even cells from other environments? The cells grown on arsenic are clearly sick—could they evolve to actually prefer arsenic to phosphorus? These are all questions that would really help us understand life on earth.
I don’t think this has the hyped impact on astrobiology. For one thing, NASA’s own definition of life scrupulously avoids mentioning specific elements as being necessary for life. Furthermore, arsenic is a much less common element in the universe than phosphorus. However, this is a really neat finding, the result of clever work, and it gives us fresh opportunity to marvel at what life can do.
Liu, Chang. C, and Peter G. Schultz. (2010). Adding New Chemistry to the Genetic Code. Annual Review of Biochemistry 79: 413-44.
Felisa Wolfe-Simon, Felisa, Jodi Switzer Blum, Thomas R. Kulp, Gwyneth W. Gordon, Shelley E. Hoeft, Jennifer Pett-Ridge, John F. Stolz, Samuel M. Webb, Peter K. Weber, Paul C. W. Davies, Ariel D. Anbar, and Ronald S. Oremland (2010). A Bacterium that can Grow by Using Arsenic Instead of Phosphorus. Published on-line ahead of print: Science DOI: 10.1126/science.1197258.
We’ll leave the last word to XKCD: (click to get the punch-line.)
Wednesday, December 1, 2010
The problem is that truth is boring
The questions the parent had were familiar to anyone who has paid attention to the vaccine "controversy." Is there a link between vaccines and autism? Is the mercury in vaccines going to cause brain damage? Is the aluminum in vaccines going to cause problems? There are so many vaccines--won't the immune system get tired out? Isn't the flu vaccine ineffective? Was the whole response to H1N1 completely overblown?
The answer to all of these questions is no. The undying persistence of these questions is really frustrating, and shows the sort of asymmetrical warfare that goes on between reality and wishful thinking. All you have to do is mention some poorly-supported hypothesis once, and it is established. If it appeals to people's fears or prejudices, then it is solidly rooted. The problem, though, is that the truth is boring. It can take a long explanation with lots of evidence and patient demonstration to prove the obvious--and even then, there has been some research showing that people still assign greater trust to the thing that they heard first, even if they've been shown solid evidence to the contrary.
So I tried to be patient and tactful with the concerned parent. I pointed out the origin of each of the rumors, how they were generally based on very incomplete and biased information. How, if you directly tested the question, the problem just disappeared. How the immune system can generate a virtually infinite number of immunities. How, due to poor sampling and a knowledge of history, H1N1 was initially terrifying to epidemiologists. How there's a whooping cough epidemic going on in California, and how it is totally unnecessary. How, if you were concerned about risk, you'd avoid McDonalds and driving. But I don't know if the concerned parent will vaccinate. Too often, if reality doesn't conform to bias, bias wins.
This pattern goes on too often. Just about any popular "debate" about science follows this pattern--evolution, climate change, vaccination, you name it. Rumor blurts something out, and refuting it takes patient work with lots of footnotes.
The day after my conversation, this cartoon appeared on the web, from the often amusing SMBC:
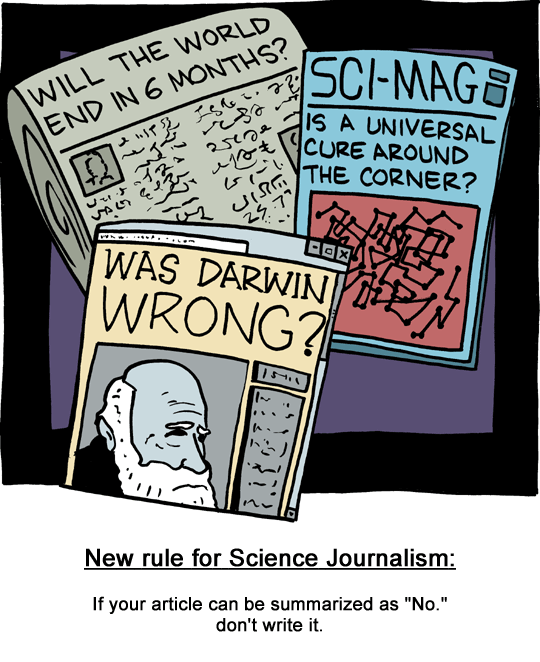